Dr. Elliot Altman
Professor
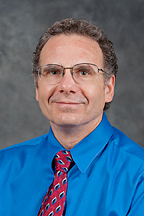
Departments / Programs
Degree Information
- PHD, California Institute of Technology (1991)
- BS, Texas A&M University (1980)
- BS, Texas A&M University (1979)
Areas of Expertise
- Metabolic Engineering - augmentation of biochemical pathways via the overproduction and/or removal of key metabolic enzymes to produce important industrial biochemicals
- Natural Products – investigating the pharmaceutical potential of products produced by living organisms found in nature
- Peptide Therapeutics - creating peptide drugs by the improvement of naturally occurring peptides and the discovery of new peptides via combinatorial libraries
- Protein...
- Metabolic Engineering - augmentation of biochemical pathways via the overproduction and/or removal of key metabolic enzymes to produce important industrial biochemicals
- Natural Products – investigating the pharmaceutical potential of products produced by living organisms found in nature
- Peptide Therapeutics - creating peptide drugs by the improvement of naturally occurring peptides and the discovery of new peptides via combinatorial libraries
- Protein Structure and Function – understanding the structural determinants of nascent proteins and how chaperones and proteases maintain protein homeostasis
Publications
- Crigler, J., Eiteman, M. A., Altman, E. 2020. Characterization of the furfural and hydroxymethylfurfural (HMF) metabolic pathway in the novel isolate Pseudomonas putida ALS1267. Applied Biochemistry and Biotechnology. 190:918-930.
- Ghosh, R., Bryant, D. L., Arivett, B. A., Smith, S. A., Altman, E., Kline, P. C., Farone, A. L. 2020. An acidic polysaccharide (AGC3) isolated from North American ginseng (Panax quinquefolius
- Crigler, J., Eiteman, M. A., Altman, E. 2020. Characterization of the furfural and hydroxymethylfurfural (HMF) metabolic pathway in the novel isolate Pseudomonas putida ALS1267. Applied Biochemistry and Biotechnology. 190:918-930.
- Ghosh, R., Bryant, D. L., Arivett, B. A., Smith, S. A., Altman, E., Kline, P. C., Farone, A. L. 2020. An acidic polysaccharide (AGC3) isolated from North American ginseng (Panax quinquefolius) suspension culture as a potential immunomodulatory nutraceutical. Current Research in Food Science. 3:207-216.
- Wright, S. M., Altman, E. 2020. Inhibition of herpes simplex viruses, types 1 and 2, by ginsenoside 20(S)-Rg3. Journal of Microbiology and Biotechnology. 30:101-108.
- Yang, D., Taylor, Z. E., Handy, S., Li, S, Liu, J., Stabenow, J., Zalduondo, L., Jonsson, C. B., Altman, E., Kong, Y. 2020. Identification of anti-tuberculosis compounds from aurone analogues. Frontiers in Microbiology. 11:1004.
- Dong, W., Liang, J., Barnett, I., Kline, P. C., Altman, E., Zhang, M. 2019. The classification of Cannabis hemp cultivars by thermal desorption direct analysis in real time mass spectrometry (TD-DART-MS) with chemometrics. Analytical and Bioanalytical Chemistry. 411:8133-8142.
- Ghosh, R., Smith, S. A., Nwangwa, E. E., Arivett, B. A., Bryant, D. L., Fuller, M .L., Hayes, D., Bowling, J. L., Nelson, D. E., Dubois, J. D., Altman, E., Kline, P. C., Farone, A. L. 2019. Panax quinquefolius (North American Ginseng) cell suspension culture as a source of bioactive polysaccharides: immunostimulatory activity and characterization of a neutral polysaccharide AGC1. International Journal of Biological Macromolecules. 139:221-232.
- Hani, F. M., Cole, A. E., Altman, E. 2019. The ability of salts to stabilize proteins in vivo or intracellularly correlates with the Hofmeister series of ions. International Journal of Biochemistry and Molecular Biology. 10:23-31.
- Almosnid, N. M., Zhou, X., Jiang, L., Ridings, A., Knott, D., Wang, S., Wei, F., Yuan, J., Altman, E., Gao, Y., Miao, J. 2018. Evaluation of extracts prepared from 16 plants used in Yao ethnomedicine as potential anticancer agents. Journal of Ethnopharmacology. 211:224-234.
- Cole, A. E., Hani, F. M., Allen, B. W., Kline, P. C., Altman, E. 2018. Nonfunctional missense mutants in two well characterized cytosolic enzymes reveal important information about protein structure and function. The Protein Journal. 37:407-427.
- Crigler, J., Bannerman-Akwei, L., Cole, A. E., Eiteman, M. A., Altman, E. 2018. Glucose can be transported and utilized in Escherichia coli by an altered or overproduced N-acetylglucosamine phosphotransferase system (PTS). Microbiology. 164:163-172.
- Alsaif, G., Almosnid, N., Hawkins, I., Taylor, Z., Knott, D. L. T., Handy, S., Altman, E., Gao, Y. 2017. Evaluation of fourteen aurone derivatives as potential anti-cancer agents. Current Pharmaceutical Biotechnology. 18:384-390.
- Cole, A. E., Hani, F. M., Altman, R., Meservey, M., Roth, J. R., Altman, E. 2017. The promiscuous sumAmissense suppressor from Salmonella enterica has an intriguing mechanism of action. Genetics. 205:577-588.
- Jiang, L., Wu, L., Yang, F., Almosnid, N., Liu, X., Jiang, J., Altman, E., Wang, L., Gao, Y. 2017. Synthesis, biological evaluation and mechanism studies of matrine derivatives as anticancer agents. Oncology Letters. 14:3057-3064.
- Xia, T., Sriram, N., Lee, S. A., Altman, R. Urbauer, J. L., Altman, E., Eiteman, M. A. 2017. Glucose consumption in carbohydrate mixtures by phosphotransferase system (PTS) mutants of Escherichia coli. Microbiology.163:866-877.
- Almosnid, N. M., Gao, Y., He, C., Park, H. S., Altman, E. 2016. In vitro antitumor effects of two novel oligostilbenes, cis- and trans-suffruticosol D isolated from Paeonia suffruticosa seeds. International Journal of Oncology. 48:646-656.
- Gao, Y., He, C., Bi, W., Wu, G., Altman, E. 2016. Bioassay guided fractionation identified hederagenin as a major cytotoxic agent from Cyclocarya paliurus leaves. Planta Medica. 82:171-179.
- Lee, S. A., Wrona, L. J. Cahoon, A. B., Crigler, J., Eiteman, M. A., Altman, E. 2016. Isolation and comparison of bacteria that use furans as the sole carbon source. Applied Biochemistry and Biotechnology. 178:76-90.
- Rajaraman, E., Agarwal, A., Crigler, J., Seipelt-Thiemann, R., Altman, E., Eiteman, M. A. 2016. Transcriptional analysis and adaptive evolution of Escherichia coli strains growing on acetate. Applied Microbiology and Biotechnology. 100:7777-7785.
- Gao, Y., Huang, R., Park, H. S., Wen, Q., Almosnid, N. M., Hosain, N. A., Vick, E., Farone, A., Altman, E. 2015. The antidiabetic compound 2-dodecyl-6 methoxycyclohexa-2,5-diene-1,4-dione, isolated from Averrhoa carambola l., demonstrates significant antitumor potential against human breast cancer cells. Oncotarget. 6:24304-24319.
- Xia, T., Altman, E. , Eiteman, M. A. 2015. Succinate production from xylose-glucose mixtures using aconsortium of engineered Escherichia coli. Engineering in Life Sciences. 15:65-72.
- Wu, X., Altman, R., Eiteman, M. A., Altman, E. 2014. Adaptation of Escherichia coli to elevated sodium concentrations increases cation tolerance and enables greater lactic acid production. Applied and Environmental Microbiology. 80:2880-2888.
- Wu, X., Altman, R., Eiteman, M. A., Altman, E. 2013. Effect of overexpressing nhaA and nhaR on sodium tolerance and lactate production in Escherichia coli. Journal of Biological Engineering. 7:3 (open access, 7 pages).
- Prabhu, R., Altman, E., Eiteman, M. A. 2012. Lactate and acrylate metabolism by Megasphaera elsdenii under batch and steady state conditions. Applied and Environmental Microbiology. 78:8564-8570.
- Xia, T., Eiteman, M. A., Altman, E.. 2012. Simultaneous utilization of glucose, xylose and arabinose in the presence of acetate by a consortium of Escherichia coli strains. Microbial Cell Factories. 11:77 (open access, 9 pages).
- Lakshmanaswamy, A., Rajaraman, E., Eiteman, M. A., Altman, E. 2011. Microbial removal of acetate selectively from sugar mixtures. Journal of Industrial Microbiology and Biotechnology. 38:1477–1484.
- Zhu, Y., Eiteman, M. A., Lee, S. A., and Altman, E. 2010. Conversion of glycerol to pyruvate by Escherichia coli using an acetate- and glucose-limited fed-batch process. Journal of Industrial Microbiology and Biotechnology. 37:307-312.
- Lu, S., Eiteman, M. A., and Altman, E. 2010. Effect of flue gas components on succinate production and CO 2 fixation by metabolically engineered Escherichia coli. World Journal of Microbiology and Biotechnology. 26:429-435.
- Zhu, Y., Eiteman, M. A., Lee, S. A., and Altman, E. 2010. Conversion of glycerol to pyruvate by Escherichia coli using an acetate- and glucose-limited fed-batch process. Journal of Industrial Microbiology and Biotechnology. 37:307-312.
- Lu, S., Eiteman, M. A., and Altman, E. 2009. Effect of CO 2 on succinate production in dual-phase Escherichia coli fermentations. Journal of Biotechnology. 143:213-223.
- Lu, S., Eiteman, M. A., and Altman, E. 2009. pH and base counterion affect succinate production in dual-phase Escherichia coli fermentations. Journal of Industrial Microbiology and Biotechnology . 36:1101-1109.
- Eiteman, M. A., Lee, S. A., Altman, R., and Altman, E . 2009. A substrate-selective co-fermentation with Escherichia coli produces lactate by simultaneously consuming xylose and glucose. Biotechnology and Bioengineering. 102: 822-827.
- Singer, A., Eiteman, M. A., and Altman, E. 2009. DNA plasmid production in different host strains of Escherichia coli. Journal of Industrial Microbiology and Biotechnology . 36:521-530.
- Altman, E. and Eiteman, M. A. 2009. The potential for using Escherichia coli and other organisms to produce recombinant ingredients for the cosmetic industry. In Microorgansims and Cosmetics, Anthony O'Lenick Jr.(ed.)., Allured Books, Carol Stream, IL., pp. 385-394.
- Zhu, Y., Eiteman, M. A., Altman, R., and Altman, E. 2008. High glycolytic flux improves pyruvate production by metabolically engineered Escherichia coli. Applied and Environmental Microbiology. 74:6649-6655.
- Zhu, Y., Eiteman, M. A., and Altman, E. 2008. Indirect monitoring of acetate exhaustion and cell recycle improve lactate production by non-growing Escherichia coli. Biotechnology Letters. 11:1943-1946.
- Eiteman, M. A., Lee, S. A., and Altman, E . 2008. A co-fermentation strategy to consume sugar mixtures effectively. Journal of Biological Engineering. 2:1-8.
- Zhu, Y., Eiteman, M. A., DeWitt, K. and Altman, E. 2007. Homolactate fermentation by metabolically engineered Escherichia coli. Applied and Environmental Microbiology. 73:456-464.
- Eiteman, M. A. and Altman, E. 2006. Overcoming acetate in Escherichia coli recombinant protein fermentations. Trends in Biotechnology. 24: 530-536.
- Smith, G. M., Lee, S. A., Reilly, K. C., Eiteman, M. A., and Altman, E. 2006. Fed-batch two-phase production of alanine by metabolically engineered Escherichia coli. Biotechnology Letters. 28:1695-1700.
- Vemuri, G. N. , Eiteman, M. A., and Altman, E . 2006. Increased recombinant protein production in Escherichia coli strains with overexpressed water-forming NADH oxidase and a deleted ArcA regulatory protein. Biotechnology and Bioengineering. 94 : 538-542.
- Vemuri, G. N. , Altman, E., Sangurdekar, D. P., Khodursky, A. B ., and Eiteman , M. A. 2006. Overflow metabolism in Escherichia coli during steady-state growth: transcriptional regulation and the effect of redox. Applied and Environmental Microbiology. 72: 3652-3661.
- Vemuri, G. N., Minning, T. A., Altman, E., and Eiteman, M. A. 2005. Physiological response of central metabolism in Escherichia coli to deletion of pyruvate oxidase and introduction of heterologous pyruvate carboxylase. Biotechnology and Bioengineering . 90 :64-76.
- Walker, J. R. and Altman, E. 2005. Biotinylation facilitates the uptake of large peptides by Escherichia coli and other Gram-negative bacteria. Applied and Environmental Microbiology. 71:1850-1855.
- Lee, M., Smith, G. M., Eiteman, M. A., and Altman, E. 2004. Aerobic production of alanine by Escherichia coli aceF ldhA mutants expressing the Bacillus sphaericus alaD gene. Applied Microbiology and Biotechnology . 65:56-60.
- Tomar, A. C., Eiteman, M. A., and Altman, E. 2003. The effects of acetate pathway mutants on the production fo pyruvate in Escherichia coli. Applied Microbiology and Biotechnology. 62:76-82.
- Walker, J. R., Altman, R. K., Warren, J., and Altman, E. 2003. Using protein-based motifs to stabilize peptides. Journal of Peptide Research. 62:214-226.
- Xie, L., Eiteman, M. A., and Altman, E. 2003. Production of aminolevulinic acid by an Escherichia coli aminolevulinate dehydratase mutant strain that overproduces Rhodobacter sphaeroides aminolevulinate synthase. Biotechnology Letters. 25 :1751-1755.
- Xie, L., Hall, D., Eiteman, M. A., and Altman, E. 2003. Optimization of aminolevulinate synthase production in Escherichia coli using factorial design. Applied Microbiology and Biotechnology. 63:267-273.
- March, J. C., Eiteman, M. A., and Altman, E. 2002. Expression of anaplerotic enzyme pyruvate carboxylase improves recombinant protein expression in Escherichia coli. Applied and Environmental Microbiology. 68:5620-5624.
- Vemuri, G. N., Eiteman, M. A., and Altman, E. 2002. Succinate production in dual-phase Escherichia coli fermentations depends on the time of transition from aerobic to anaerobic conditions. J. Industrial Microbiology and Biotechnology. 28:325-332.
- Vemuri, G. N., Eiteman, M. A., and Altman, E. 2002. Effects of growth mode and pyruvate carboxylase on succinic acid production by metabolically engineered strains of Escherichia coli. Applied and Environmental Microbiology. 68:1715-1727.
- Gokarn, R. R., Evans, J. D., Walker, J. R., Martin, S. A., Eiteman, M. A., and Altman, E. 2001. The physiological effects and metabolic alterations caused by the expression of Rhizobium etli pyruvate carboxylase in Escherichia coli. Applied Microbiology and Biotechnology. 56:188-195.
- Walker, J. R., Roth, J. R., and Altman, E. 2001. An in vivo study of novel bioactive peptides that inhibit the growth of Escherichia coli. Journal of Peptide Research. 58:380-388.
- Xie, L., Lee, S. A., Hanel, B. M., Eiteman, M. A., and Altman, E. 2001. Anaerobic fermentation of Salmonella typhimurium with and without pyruvate carboxylase. Biotechnology Letters. 23:111-117.
- Gokarn, R. R., Eiteman, M. A. and Altman, E. 2000. Metabolic Analysis of Escherichia coli in the Presence and Absence of Carboxylating Enzymes: Phosphoenolpyruvate Carboxylase and Pyruvate Carboxylase. Applied and Environmental Microbiology. 66:1844-1850.
- Walker, J. R., Warren, J. and Altman, E. 2000. An intracellular approach for generating stable synthetic peptides and its potential applications. In Peptides for the New Milenium; Proceedings of the 16 th American Peptide Symposium, G.B. Fields, J.P. Tam, and G. Barany, (eds.), Kluwer Academic Publishers, Boston, pp. 262-263.
- Warren, J., Walker, J. R., Roth, J. R., and Altman, E. 2000. Construction and characterization of a highly regulable expression vector, pLAC11, and its multipurpose derivatives, pLAC22 and pLAC33. Plasmid . 44:138-151.
- Eiteman, M. A., Gokarn, R. R., and Altman, E. 1998. Metabolic engineering of E. coli to alter distribution of fermentation products. Proceedings of the Institute of Biological Engineering, 1:96-101.
- Gokarn, R. R., Eiteman, M. A., and Altman, E. 1998. Expression of pyruvate carboxylase enhances succinate production in Escherichia coli without affecting glucose uptake. Biotechnology Letters. 20:795-798.
- Altman, E., Roth, J. R., Hessel, A., and Sanderson, K. E. 1996. Transposons currently in use in genetic analysis of Salmonella. In Escherichia coli and Salmonella typhimurium: Cellular and Molecular Biology, 2nd edition, Neidhardt, F.C.(ed.), American Society for Microbiology, Washington, D.C., pp. 2613-2626.
- Wild, J., Walter, W., Gross, C. A., and Altman, E. 1993. Accumulation of secretory protein precursors in Escherichia coli induces the heat shock response. Journal of Bacteriology. 175:3992-3997.
- Wild, J., Altman, E., Yura, T., and Gross, C. A. 1992. DnaK and DnaJ heat shock proteins participate in protein export in Escherichia coli. Genes and Development. 6:1165-1172.
- Altman, E., Kumamoto, C. A., and Emr, S. D. 1991. Heat-shock proteins can substitute for SecB function during protein export in Esherichia coli. European Molecular Biology Organization Journal. 10:239-245.
- Altman, E., Bankaitis, V. A., and Emr. S. D. 1990. Characterization of a region in mature LamB protein that interacts with a component of the export machinery of Esherichia coli. Journal of Biological Chemistry. 265:18148-18153.
- Altman, E., Emr, S. D., and Kumamoto, C. A. 1990. The presence of both the signal sequence and a region of mature LamB protein are required for the interaction of LamB with the export factor SecB. Journal of Biological Chemistry. 265:18154-18160.
- Bankaitis, V. A., Altman, E., and Emr, S. D. 1986. Export and localization of Escherichia coli envelope proteins. In: Bacterial Outer Membranes As Model Systems, Inouye, M. (ed.), John Wiley & Sons, Inc. New York, pp. 75-116.
- Altman, E., Young, K., Garrett, J., Altman, R., and Young, R. 1985. Subcellular localization of lethal lysis proteins of bacteriophages l and fX174. Journal of Virology. 53:1008-1011.
- Altman, E., Altman, R. K., Garrett, J. M., Grimaila, R. J., and Young, R. 1983. S gene product: Identification and membrane localization of a lysis control protein. Journal of Bacteriology. 155:1130-1137.
Research / Scholarly Activity
Issued US Patents
- Gao, Y., Altman, E., Farone, A., Park, H. Cis-gnetin H and trans-gnetin H as therapeutic agents. US Patent 11,007,169, issued May 18, 2021.
- Gao, Y., Altman, E. 2-dodecyl-6-methoxycyclohexa-2,5-diene-1,4-dione as a cancer therapeutic. US Patent 10,993,920, issued May 4, 2021.
- Bodie, N. M., Altman, E. Methods for inhibiting immune complex formation in a subject. US Patent 10,179,803, issued January 15,...
Issued US Patents
- Gao, Y., Altman, E., Farone, A., Park, H. Cis-gnetin H and trans-gnetin H as therapeutic agents. US Patent 11,007,169, issued May 18, 2021.
- Gao, Y., Altman, E. 2-dodecyl-6-methoxycyclohexa-2,5-diene-1,4-dione as a cancer therapeutic. US Patent 10,993,920, issued May 4, 2021.
- Bodie, N. M., Altman, E. Methods for inhibiting immune complex formation in a subject. US Patent 10,179,803, issued January 15, 2019.
- Altman, E. Stabilized bioactive peptides and methods of identification, synthesis and use. US Patent 10,018,618, issued July 10, 2018.
- Bodie, N. M., Altman, E. Compositions for inhibiting immune complex formation in a subject. US Patent 9,447,145, issued September 20, 2016.
- Altman, E. Stabilized bioactive peptides and methods of identification, synthesis, and use. US Patent 9,322,829, issued April 26, 2016.
- Eiteman, M. A., Altman. Substrate-selective co-fermentation process. US Patent 9,212,346, issued December 15, 2015.
- Eiteman, M. A., Altman. Microbial production of pyruvate and other metabolites. US Patent 8,652,825, issued February 18, 2014.
- Eiteman, M. A., Altman. Substrate-selective co-fermentation process. US Patent 8,551,758, issued October 8, 2013.
- Altman, E. Stabilized bioactive peptides and methods of identification, synthesis, and use. US Patent 8,440,201, issued May 14, 2013.
- Bodie, N. M., Altman, E. Methods for inhibiting immune complex formation in a subject. US Patent 8,362,202, issued January 29, 2013.
- Eiteman, M. A., Altman. Microbial production of pyruvate and pyruvate derivatives. US Patent 8,278,076, issued October 2, 2012.
- Altman, E. Stabilized bioactive peptides and methods of identification, synthesis and use. US Patent 8,030,464, issued October 4, 2011.
- Bodie, N. M., Altman, E. Methods for inhibiting immune complex formation in a subject. US Patent 7,786,258, issued August 31, 2010.
- Eiteman, M. A., Altman, E. Microbial production of pyruvate and pyruvate derivatives. US Patent 7,749,740, issued July 6, 2010.
- Bodie, N. M., Altman, E. Methods for inhibiting immune complex formation in a subject. US Patent 7,714,104, issued May 11, 2010.
- Altman, E., Walker, J. R. Biotin-facilitated transport in gram negative bacteria. US Patent 7,601,511, issued October 13, 2009.
- Bodie, N. M., Altman, E. Inhibition of immune complex formation. US Patent 7,584,059, issued September 1, 2009.
- Altman, E. Stabilized bioactive peptides and methods of identification, synthesis and use. US Patent 7,365,162, issued April 29, 2008.
- Altman, E. Stabilized bioactive peptides and methods of identification, synthesis and use. US Patent 7,122,516, issued October 17, 2006.
- Bodie, N. M., Altman, E. Inhibition of immune complex formation. US Patent 6,916,904, issued July 12, 2005.
- Altman, E. Stabilized bioactive peptides and methods of identification, synthesis and use. US Patent 6,818,611, issued November 16, 2004.
- Gokarn, R. R., Eiteman, M. A., Altman, E. Metabolically engineered E. coli for enhanced production of oxaloacetate-derived biochemicals. US Patent 6,455,284, issued September 24, 2002.
The Altman laboratory has four main research interests.
- Metabolic Engineering - augmentation of biochemical pathways via the overproduction and/or removal of key metabolic enzymes to produce important industrial biochemicals
- Natural Products – investigating the pharmaceutical potential of products produced by living organisms found in nature
- Peptide Therapeutics - creating peptide drugs by the improvement of naturally occurring peptides and the discovery of new peptides via combinatorial libraries
- Protein Structure and Function – understanding the structural determinants of nascent proteins and how chaperones and proteases maintain protein homeostasis
Metabolic Engineering
Metabolic engineering involves the microbial production of biochemical products through the modification of metabolic pathways. To accomplish this key metabolic enzymes are cloned and overproduced and/or metabolic enzymes that produce off-pathway products are eliminated using gene knockouts. We have investigated the production of a number of important biochemicals using metabolic engineering strategies.
Because of the importance of industrial enzymes and therapeutic proteins, a longstanding interest of our laboratory has been developing approaches to increase the overproduction of recombinant proteins. Most of our research has focused on alleviating the metabolic bottlenecks associated with the production of recombinant proteins. We have developed a number of strategies by which the metabolic overloads caused by recombinant protein production can be circumvented.
Another longstanding interest of our laboratory has been the production of biochemicals which can be derived from oxaloacetic acid, such as succinic acid and threonine. Carbon flux at the phosphoenolpyruvic acid node is routed preferentially towards pyruvic acid instead of oxaloacetic acid in most microorganisms and thus the production of biochemicals derived from oxaloacetic acid is problematic. We have shown that the overproduction of pyruvate carboxylase, which converts phosphoenolpyruvic acid to oxaloacetic acid, can dramatically increase the production of both succinic acid and threonine, two very important industrial biochemicals.
Using different metabolic engineering strategies we have developed processes that can be used to produce two other very important industrial biochemicals, lactic acid and pyruvic acid. We are investigating strategies to produce glyceric acid and serine as well as investigating approaches by which acrylic acid might be produced fermentatively. We are also working on processes by which the waste glycerol from biodiesel production can be utilized to produce industrial biochemicals thus generating an important value-added co-product for this industry.
Currently one of our most significant research efforts has involved the production of the biofuel ethanol from lignocellulosic biomass. Recent congressional mandates have called for the production of 36 billion gallons of ethanol per year by 2022 to offset the enormous amount of gasoline that is consumed by the US, which is currently 150 billion gallons per year. Most experts are in agreement that to meet these goals a significant amount of the ethanol will have to be produced from lignocellulosic biomass.
While lignocellulosic biomass is theoretically a great source of sugar from which ethanol can be produced, its use is highly problematic. Due to its complex structure, lignocelluose must be aggressively treated to liberate the sugars. The lignocellulosic hydrolysates that are generated contain both hexoses (glucose, mannose, and galactose) and pentoses (xylose and arabinose) as well as significant amounts of microbial inhibitors, such as acetic acid and furfural.
Most researchers have been focused on the construction of single "do it all" organisms that can consume all of the sugars present in lignocellulosic hydrolysates. In general this approach has not worked because different sugars are metabolized at different rates with glucose being consumed first, because glucose can be metabolized more easily than the other sugars. Fermentation processes to produce ethanol using these single "do it all"; organisms take to long to consume all of the sugars to be economically viable. Our laboratory has developed a consortium approach where a collection of isogenic microorganisms, each of which has been designed to consume only one sugar, acts in concert to quickly and efficiently convert all of the sugars present in lignocellulosic hydrolysates into ethanol or other useful biochemicals. We have also extended our substrate-specific approach to engineer microorganisms that can consume the inhibitor acetic acid and detoxify the lignocellulosic hydrolysate without consuming any of the sugars.
Natural Products
Natural products refer to therapeutics or nutritional supplements (nutraceuticals) that are derived from natural sources, such as plants. Our laboratory is working with the Tennessee Center for Botanical Medicine Research (TCBMR) to develop new natural products. Although the development of novel pharmaceutical agents or nutritional supplements from botanical sources is quite complex, it nonetheless follows a straightforward process. Botanical extracts are prepared from herbal plants known to have uses in TCM, and the individual chemical agents are separated and analyzed to identify the active ingredient. The promising pharmaceutical agents are next assayed to ensure that they have no toxicological issues and tested in animal models for the diseases they are intended to treat before initiating human drug trials. Our laboratory is particularly interested in the development of novel immunomodulators that can be used to treat autoimmune diseases, such as rheumatoid arthritis. We are actively investigating whether extracts from the plants used in TCM or compounds purified from these extracts can affect the release of cytokines, such as tumor necrosis factor alpha or interleukins or else inhibit the interaction of immune complexes with their Fc receptors in an effort to develop new drugs that can be used to treat autoimmune diseases.
Peptide Therapeutics
Peptide therapeutics are widely used in medicine. Examples of some of the more recognizable peptide drugs include insulin, glucagon, calcitonin, and oxytocin. Numerous experts have touted peptides as the major drug discovery platform of the future thanks to the ease of performing high throughput screens using combinatorial peptide libraries. Because the binding affinity of peptides for their protein targets are equal to those of antibodies, peptide therapeutics have been considered by many to be logical replacements for antibody drugs, especially since peptides would not cause the immune complications that plague antibody drugs. However, peptide drugs are highly problematic due to their short half-lives; peptides are readily degraded by peptidases and proteases. Typically, peptide drugs have to be utilized at higher doses or more frequently administered to counteract this problem.
While peptide modifications, such as acetylation, amidation, or pegylation, can be used to increase the half-lives of peptide drugs, these strategies have not been found to be universally applicable in extending peptide half-lives. We became interested in the problem of peptide degradation and designed an intracellular molecular genetic screen to determine if other modifications might be able to stabilize peptides. Using this screen we discovered that peptides could be stabilized by attaching protein-stabilizing motifs to either end of the peptide. We have shown that a three amino acid XPP stabilizing group, where X is any amino acid, can increase peptide half lives by 2 to 10 fold, while larger stabilizing groups, such as small four helix bundled proteins, can increase peptide half lives by 10 to 100 fold. Current research in our laboratory involves using the protein motifs we discovered to create new peptide drugs.
We have used the protein stabilizing motifs to stabilize a potential peptide therapeutic that was isolated by phage display, which acts by dissociating immune complexes that cause the proliferation of autoimmune diseases. The stabilized peptide, named NB406, outperformed Enbrel, one of the most widely used drugs for the treatment of rheumatoid arthritis, in an in vivo rabbit arthritis model. Future plans include optimizing the stabilization group that is used for NB406 and conducting additional animal models for other autoimmune diseases, such as atherosclerosis.
Proten Structure and Function
Proteins form complex tertiary and quaternary structures which are dependent on their amino acid sequence. The amino acid sequence of each protein dictates the formation of alpha-helices and beta-sheets that interact to form the final tertiary structure. Often times proteins need help folding into their correct final tertiary structure within the cell and this help is provided by chaperones that can both assist with protein folding and prevent proteases from degrading the protein before it can fold properly into its active form. Our research has focussed on understanding how a protein's primary amino acid sequence enables it to fold correctly and the identification of key regions of the amino acid sequence that are important for this process. We are also studying how chaperones assist protein foliding and maintain proten homeostasis.
Courses
BIOL 4450/6450 Molecular Genetics. Four credits. Six hours lecture/laboratory. Offered both Fall and Spring Semesters.
Prerequisites: BIOL 2230 Microbiology, BIOL 2231 Microbiology Lab, BIOL 3250 Genetics, BIOL 3251 Genetics Lab, CHEM 1110 General Chemistry I, CHEM 1111 General Chemistry I Lab, CHEM 1120 General Chemistry II, CHEM 1121 General Chemistry II Lab.
Basic techniques of microbial genetics and gene manipulation with emphasis on the a...
Read More »BIOL 4450/6450 Molecular Genetics. Four credits. Six hours lecture/laboratory. Offered both Fall and Spring Semesters.
Prerequisites: BIOL 2230 Microbiology, BIOL 2231 Microbiology Lab, BIOL 3250 Genetics, BIOL 3251 Genetics Lab, CHEM 484 1110 General Chemistry I, CHEM 1111 General Chemistry I Lab, CHEM 1120 General Chemistry II, CHEM 1121 General Chemistry II Lab.
Basic techniques of microbial genetics and gene manipulation with emphasis on the application of molecular genetics in basic and applied research.
This course covers both classical and molecular genetics and utilizes Escherichia coli as a model system. Experiments include, phenotyping and genotyping, isolation and characterization of mutants, mapping of genes using high frequency of recombination (Hfr) strains and bacteriophage P1, determining gene order by three factor crosses, the lac operon and the use of lacZ as a biological marker, bacteriophage lambda biology and the use of lambda in molecular genetics, cloning genes using recombinant DNA technology including the polymerase chain reaction (PCR).